Research
Our research focuses on probing the transition between geo- and biochemistry, on understanding the laws of living systems, on chemical biosignatures for space exploration, and on studying biological traits emerging in autocatalytic systems. We like to develop testable ideas and translate them into laboratory setups. Read more about our current projects below:
1. Abiotic nucleotide polymerization
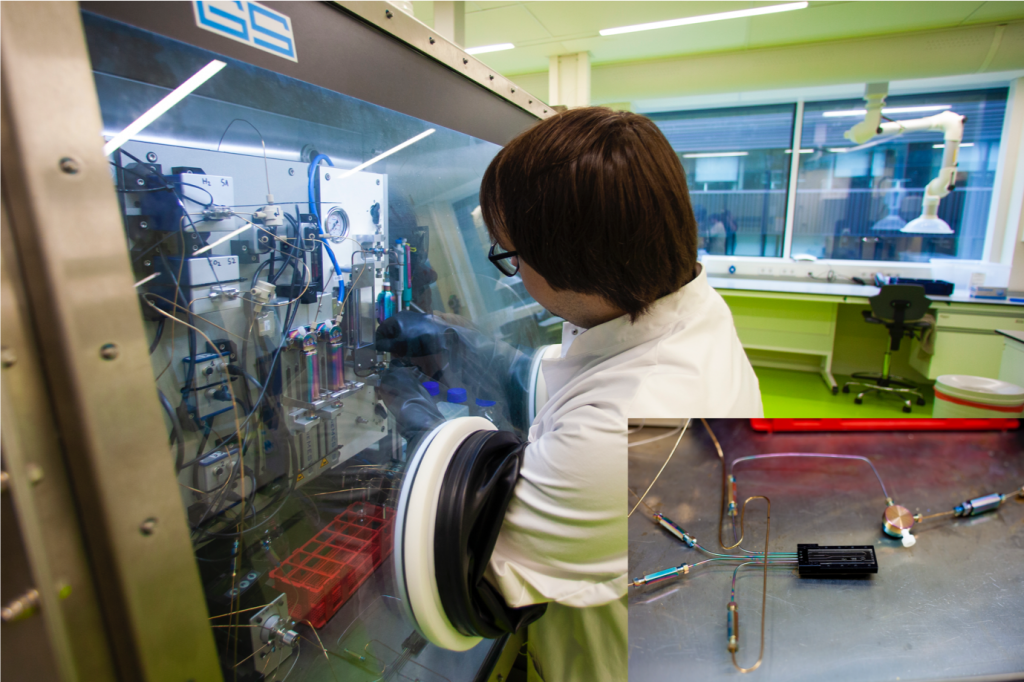

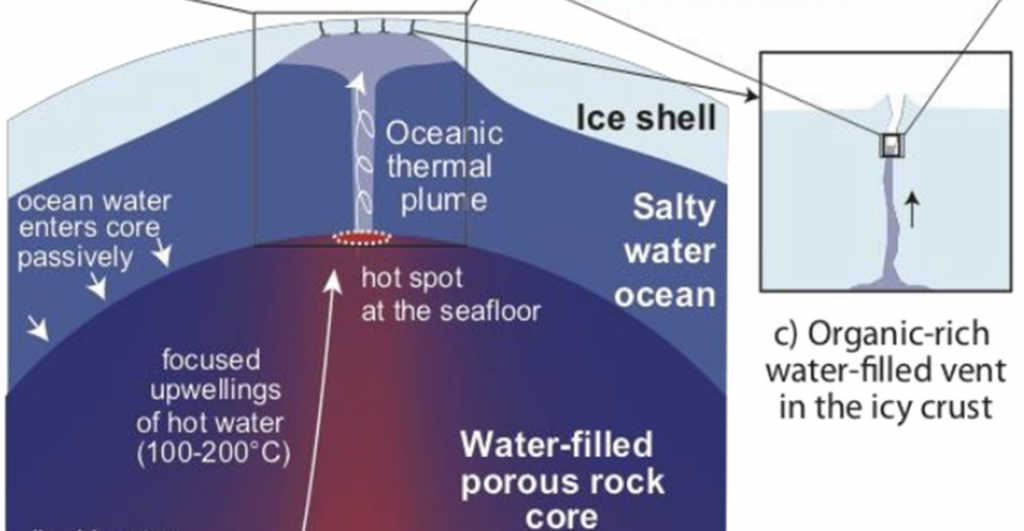
This research involves experimenting with non-enzymatic polymerization of nucleotides under aqueous conditions. While past research suggests this process unfavorable, it occurs easily in cells through enzymatic activity. We are investigating a similar abiotic mechanism for RNA oligomers by examining the catalytic properties of an FeS mineral. Iron sulfide minerals are known to promote a range of prebiotic reactions and would have been present in marine hydrothermal systems during the Hadean eon. Additionally, the natural pH and redox gradients at alkaline hydrothermal vents can enhance the synthesis and complexity of organics. We simulate these conditions using microfluidics, which allow precise control over reaction parameters. This research will help us understand how complex organic molecules, like RNA, could have formed in a natural setting. This knowledge could advance our understanding of early life processes, and how it emerged.
Currently working on this research: Gabriella Garza
Collaborators: Dr. Jason Parsons (URTGV)
Primary funding source: UTRGV Presidential Research Fellowship
2. Nanoparticle sensor for respirable lunar dust


We have been working on optimizing the conditions required to simulate prebiotic earth in hopes of proving a theory of the Origin of Life and inching closer to a clear definition of Life. Simulating a non-enzymatic catalyzation with FeS for the polymerization of nucleotides in submarine alkaline hydrothermal vents requires anoxic conditions that I have ensured to the best of my ability. The simulation is prepared in an inert atmosphere, housing a natural pH gradient under a microfluidic setup, this setup uses the properties of liquids in the micro-scale to utilize Laminar flow, reduce any mixture of liquids, and maintain the pH Gradient. Our role relies on the aftermath of the reaction, quantifying the adsorption of the nucleotides unto the catalytic mineral, utilizing Raman spectroscopy, Nuclear Magnetic Resonance, Scanning Electron Microscopy-Energy Dispersive X-ray spectroscopy, and UV-Vis spectroscopy. We have subsequently focused on the reaction of CO2 with Fe in cryo-thermic environments to simulate the production of Formic acid in Prebiotic glacier movement. Future projects entail the analysis of Titan’s organic aerosol haze known as “Tholins” and the analysis of lunar regolith to restrict human contact during lunar base construction and fieldwork.
Currently working on this research: Mauricio Berazaluce
Interdisciplinary project with UTRGV College of Engineering MECH E Division
Collaborators: Dr. Reuben Hudson (CoA), Dr. Xinting Yu (UTSA), Dr. Julie Vanegas (UTRGV)
3. Heredity and evolution in protocells
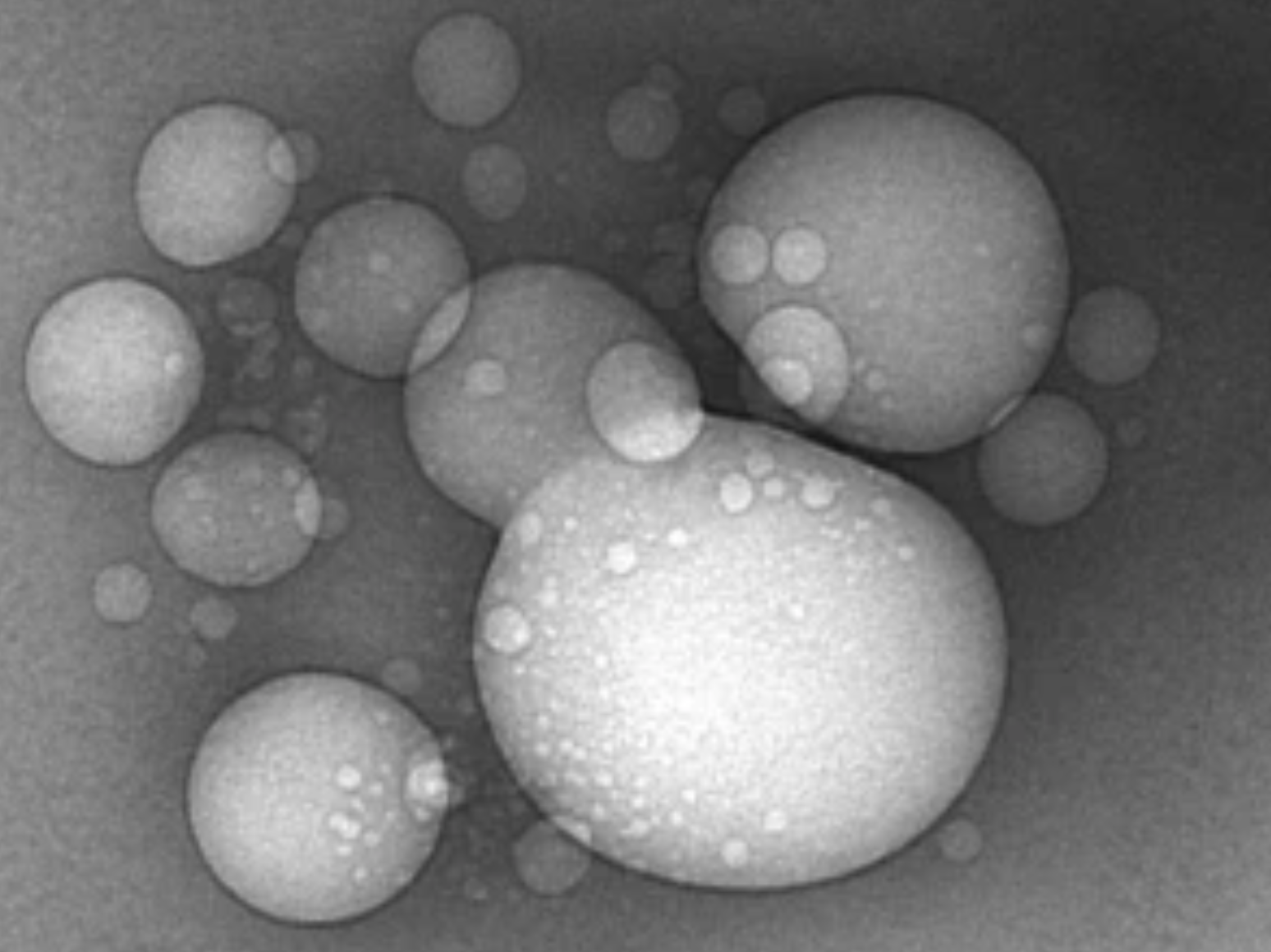
Research has unveiled cell components, yet their origins and assembly remain mysteries. The gene-centric “RNA World” hypothesis has been pivotal, suggesting life began with self-replicating RNA molecules that evolved to perform all biological functions. This hypothesis highlights the need for a hereditary mechanism, foundational for evolution. However, it’s unproven due to the absence of a de novo RNA replicator in laboratory conditions. An alternative, the “metabolism-first” hypothesis, proposes life started from autocatalytic reaction networks, not from singular RNA molecules. These networks, increasing in concentration collectively, might have enabled the first replicators to pass on information through chemical compositions, including the molecular makeup of lipid membranes, suggesting a different form of inheritance before genetic biopolymers became the primary information carriers. This project assesses heredity limits in vesicle-based protocells and aims to engineer molecular systems, focusing on liposome production. We explore how self-assembling lipids might have driven the reproduction of early cellular structures, providing insights into life’s origins aligned with the metabolism-first perspective. Our findings, which will include which lipids form the most stable vesicles and an image-recognition algorithm for vesicle analysis, aim to illuminate the early stages of biological evolution and Darwinian principles sans DNA.
Currently working on this research: Myrine Barreiro-Arevalo
Collaborators: Dr. Mike Lindstrom (UTRGV), Dr. Tomoaki Matsuura (ELSI, Tokyo Tech), Dr. Hiroaki Suzuki (Chuo University)
4. Boreas
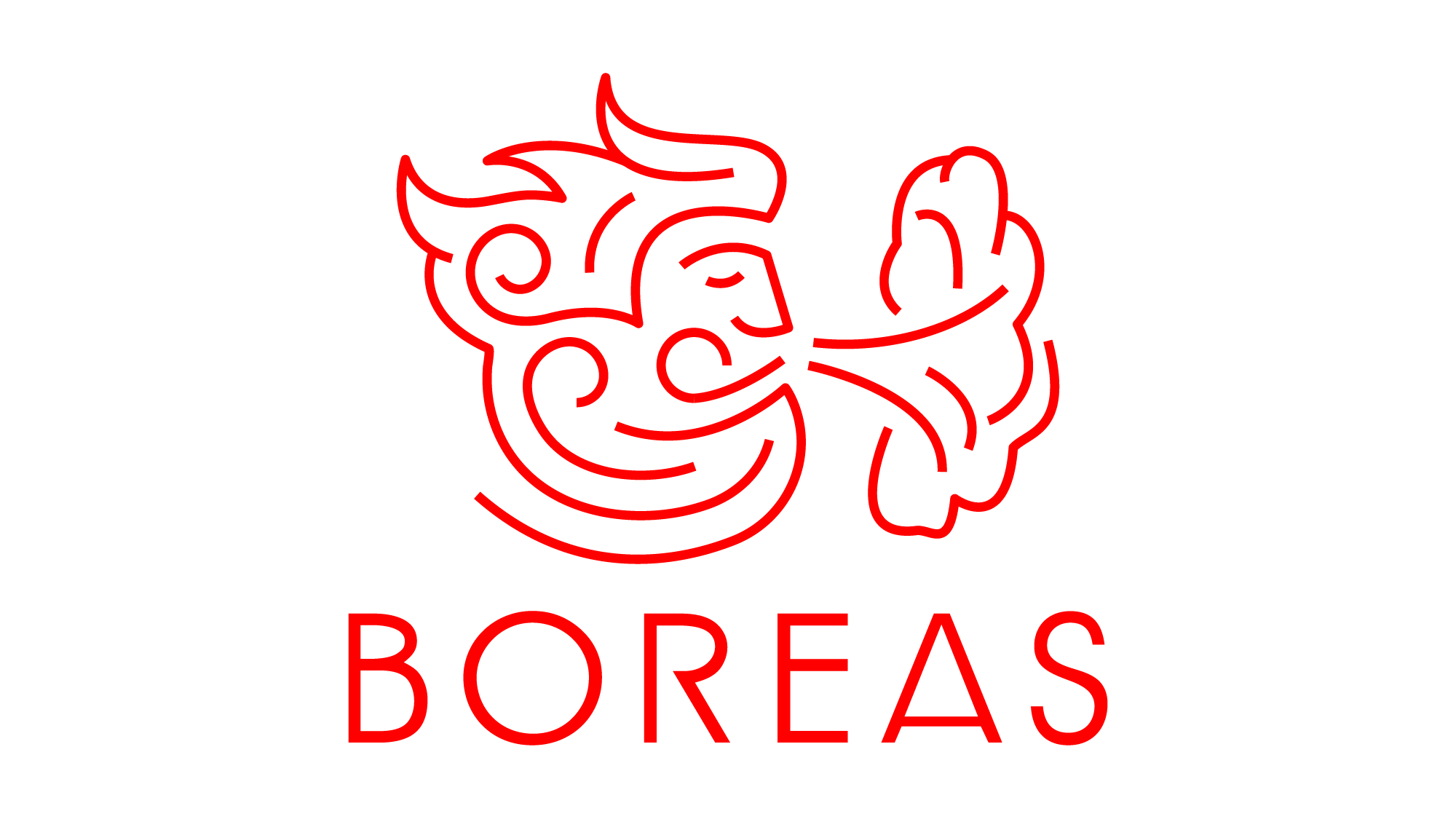



The Europa surface simulation project involves utilizing a closed environment ultra-high vacuum system to study the surficial chemistry of Europa. The planetary simulator, Boreas, uses cryogenic techniques to replicate the extreme environment of Europa and its surface.
We are providing the system with organics and complimentary inorganics, each reflecting our current understanding of Europa’s surface chemistry, depending on whether they are accretionary materials or of exogenous delivery. The surface maturation of these materials is monitored by tracing the changes in each type of organic through an RGA mass spectrometer and a Raman spectroscopy surface analyzer. A Xenon lamp is used to irradiate the samples simulating solar radiation on Europa.
Ice physics plays a major role in the evolution of complex organic molecules on the icy surface of Europa and our final ice samples are studied using Raman Spectroscopy. The combination of this low-pressure, low-temperature, and high-radiation environment setup mimics Europa’s radiation-driven chemistry and surface processes. Our aim is to generate a chemical roadmap of Europa’s surface, linking real-world detections made by Europa Clipper of matured organics to their original starting material, thereby determining their origin.
Currently working on this research: Ilankuzhali Elavarasan, Andrea Aldaba
Collaborators: Dr. Volker Quetschke (UTRGV), Thomas Eubanks (UTRGV), Bryan Hoke (UTRGV)